Define Order And Molecularity Of A Reaction
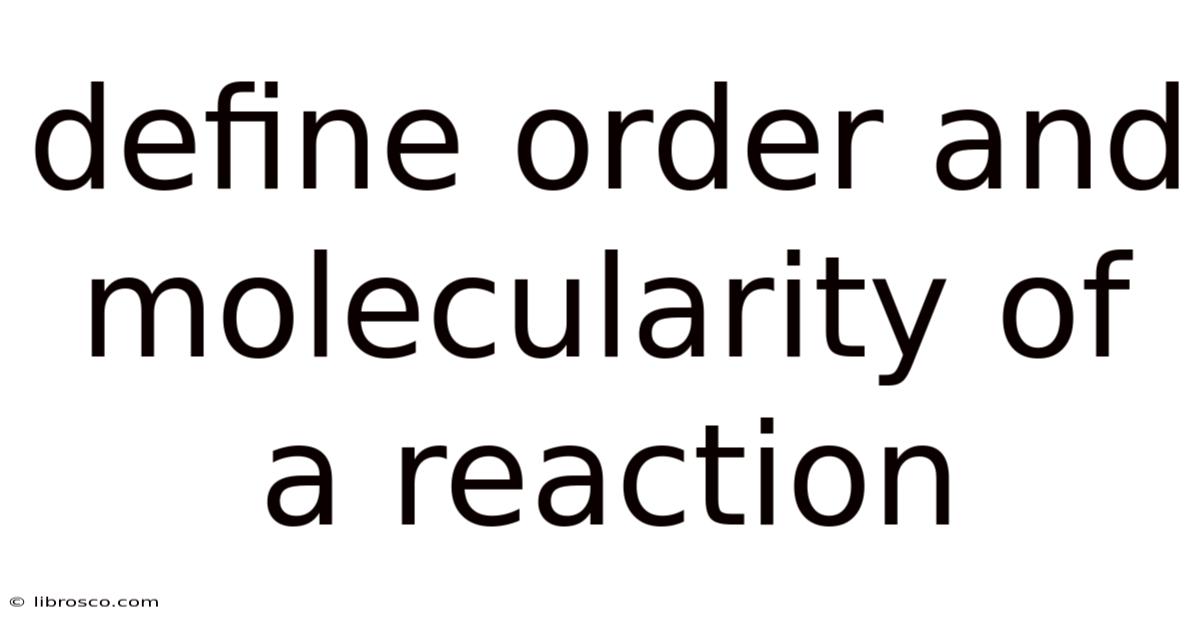
Discover more detailed and exciting information on our website. Click the link below to start your adventure: Visit Best Website meltwatermedia.ca. Don't miss out!
Table of Contents
Defining Order and Molecularity of a Reaction: Unlocking the Secrets of Chemical Kinetics
What if a deeper understanding of reaction order and molecularity could unlock the secrets to optimizing chemical processes and designing novel materials? These fundamental concepts are the cornerstone of chemical kinetics, providing crucial insights into reaction rates and mechanisms.
Editor’s Note: This article on defining reaction order and molecularity was published today, offering current and accurate information on this vital area of chemistry. It provides a comprehensive overview for students and professionals seeking a clearer understanding of these key concepts.
Why Reaction Order and Molecularity Matter: Relevance, Practical Applications, and Industry Significance
Reaction order and molecularity are not merely abstract concepts; they are essential tools for understanding and controlling chemical reactions. In numerous industries, from pharmaceuticals and materials science to environmental engineering and catalysis, manipulating reaction rates is crucial for efficiency, safety, and product quality. A thorough grasp of these concepts allows chemists and engineers to design reactors, optimize reaction conditions, and predict reaction outcomes with greater accuracy. For instance, understanding the order of a reaction helps in determining the appropriate catalyst concentration or temperature to achieve desired reaction rates. Similarly, knowledge of molecularity aids in proposing plausible reaction mechanisms and understanding the steps involved in a complex process.
Overview: What This Article Covers
This article provides a comprehensive exploration of reaction order and molecularity. We will define these terms, differentiate them clearly, explore different types of reaction orders, illustrate their determination through experimental data, and delve into the relationship between molecularity and reaction mechanism. The article also examines the complexities arising from multi-step reactions and provides practical examples to enhance understanding.
The Research and Effort Behind the Insights
This article draws upon established principles of chemical kinetics, incorporating information from numerous textbooks, research papers, and reputable online resources. The explanations are supported by illustrative examples and mathematical formulations, ensuring clarity and accuracy. A structured approach has been employed to present the information logically and systematically.
Key Takeaways:
- Definition and Core Concepts: A clear distinction between reaction order and molecularity.
- Determination of Reaction Order: Methods for experimentally determining reaction order.
- Molecularity and Reaction Mechanisms: The connection between molecularity and the elementary steps of a reaction.
- Complex Reactions and Rate Laws: Handling reactions involving multiple steps.
- Practical Applications: Examples of how reaction order and molecularity are used in real-world applications.
Smooth Transition to the Core Discussion:
Having established the importance of reaction order and molecularity, let's now delve into a detailed exploration of each concept.
Exploring the Key Aspects of Reaction Order and Molecularity:
1. Definition and Core Concepts:
Reaction order: This refers to the experimentally determined relationship between the rate of a reaction and the concentration of reactants. It is an empirical quantity obtained from experimental data and does not necessarily reflect the stoichiometry of the reaction. The order with respect to a particular reactant is the exponent to which the concentration of that reactant is raised in the rate law. For example, a reaction with a rate law of Rate = k[A]²[B] is second order with respect to A, first order with respect to B, and third order overall.
Molecularity: This describes the number of molecules (or atoms or ions) that participate directly in an elementary reaction (a single-step reaction). Molecularity can only be applied to elementary reactions, unlike reaction order which applies to overall reactions, regardless of mechanism. An elementary reaction can be unimolecular (one molecule involved), bimolecular (two molecules involved), or termolecular (three molecules involved). Termolecular reactions are relatively rare due to the low probability of three molecules simultaneously colliding with the correct orientation and energy.
2. Determination of Reaction Order:
Reaction order is typically determined experimentally by monitoring the concentration of reactants over time under various conditions. Common methods include:
-
Method of Initial Rates: This involves measuring the initial rate of the reaction at different initial concentrations of reactants. By comparing the changes in initial rates with corresponding changes in concentration, the order with respect to each reactant can be determined.
-
Graphical Method: This involves plotting the concentration of a reactant versus time. The order of the reaction can be inferred from the shape of the curve. For example, a first-order reaction yields a straight line when ln[A] is plotted against time, while a second-order reaction yields a straight line when 1/[A] is plotted against time.
-
Half-life Method: The half-life (t₁/₂) of a reaction is the time it takes for half of the reactant to be consumed. For first-order reactions, the half-life is independent of the initial concentration, while for second-order reactions, the half-life is inversely proportional to the initial concentration.
3. Molecularity and Reaction Mechanisms:
The molecularity of an elementary reaction provides insights into the reaction mechanism. The mechanism is a step-by-step description of how the reactants are transformed into products. For example, a unimolecular reaction might involve a molecule rearranging itself, while a bimolecular reaction might involve two molecules colliding and reacting. However, it's important to note that the overall order of a reaction doesn't directly correspond to the molecularity of each step in a complex multi-step reaction.
4. Complex Reactions and Rate Laws:
Many reactions occur via a series of elementary steps. The overall rate law for a complex reaction is not simply determined by adding the molecularities of the individual steps. Instead, the rate-determining step (the slowest step) often dictates the overall rate law. The rate law must be determined experimentally, even for complex reactions, as theoretical predictions based on molecularity alone may be inaccurate.
5. Practical Applications:
Understanding reaction order and molecularity is crucial in various applications:
-
Reactor Design: The design of chemical reactors (e.g., batch, continuous flow) is heavily influenced by the reaction order. The choice of reactor type and operating conditions depend on the desired reaction rate and conversion.
-
Catalysis: Catalysts can significantly alter reaction rates by modifying the reaction mechanism. Knowing the reaction order allows for the optimization of catalyst concentration and type.
-
Pharmaceutical Development: The kinetics of drug metabolism and drug interactions are critically dependent on reaction order.
-
Environmental Chemistry: Understanding reaction orders helps in predicting the fate of pollutants in the environment and developing effective remediation strategies.
Exploring the Connection Between Reaction Intermediates and Reaction Order
Reaction intermediates are species that are formed in one elementary step of a reaction and consumed in a subsequent step. They are not present in the overall stoichiometric equation. The presence of reaction intermediates often complicates the relationship between molecularity and overall reaction order. For example, a multi-step reaction with a fast initial step forming an intermediate followed by a slower rate-determining step involving that intermediate will have an overall reaction order determined by the slower step, not the molecularity of the faster step involving the intermediate's formation. The concentration of the intermediate itself is usually difficult to measure directly, which adds another layer of complexity to determining the overall reaction order.
Key Factors to Consider:
-
Roles and Real-World Examples: In enzymatic reactions, for instance, the enzyme acts as a catalyst and forms a temporary intermediate complex with the substrate. The rate-determining step might involve the breakdown of this complex, which influences the overall reaction order.
-
Risks and Mitigations: Incorrectly assuming that the overall reaction order is equal to the sum of the stoichiometric coefficients can lead to inaccurate predictions and inefficient reaction design. Careful experimental determination of the reaction order is essential to mitigate this risk.
-
Impact and Implications: Accurate determination of reaction order is critical for designing efficient and safe chemical processes. It enables precise control of reaction rates, optimal resource utilization, and improved product yield.
Conclusion: Reinforcing the Connection
The interplay between reaction intermediates and reaction order highlights the importance of experimentally determining rate laws instead of relying solely on stoichiometry or the molecularity of individual steps. A thorough understanding of this connection is vital for accurate prediction and control of reaction behavior.
Further Analysis: Examining Reaction Mechanisms in Greater Detail
A detailed understanding of a reaction's mechanism is crucial for predicting its order. While the overall reaction order can be experimentally determined, the mechanism needs to be proposed and tested through various experiments. Techniques like isotopic labeling, kinetic isotope effects, and spectroscopic analysis are instrumental in elucidating reaction mechanisms, confirming proposed intermediates, and understanding the steps involved. By studying the mechanism, one can gain insight into the rate-determining step, which then helps correlate the observed reaction order with the molecularity of the rate-determining step. This detailed mechanistic understanding allows for better control and optimization of the chemical reaction.
FAQ Section: Answering Common Questions About Reaction Order and Molecularity
Q: What is the difference between reaction order and molecularity?
A: Reaction order is an experimentally determined quantity reflecting the relationship between reactant concentrations and reaction rate. Molecularity describes the number of molecules participating in an elementary reaction step. Reaction order applies to overall reactions, while molecularity applies only to elementary reactions.
Q: Can a reaction have a fractional order?
A: Yes, fractional orders are possible and often indicate complex mechanisms involving multiple steps.
Q: How can I determine the reaction order experimentally?
A: The method of initial rates, graphical methods, and the half-life method are commonly used to determine reaction order experimentally.
Q: What is the significance of the rate-determining step?
A: The rate-determining step is the slowest step in a multi-step reaction; it dictates the overall reaction rate and, consequently, the observed reaction order.
Practical Tips: Maximizing the Benefits of Understanding Reaction Order and Molecularity
-
Conduct Thorough Experiments: Always determine reaction order experimentally using reliable methods.
-
Understand Reaction Mechanisms: Attempt to propose a plausible reaction mechanism to explain the observed reaction order.
-
Consider Complexities: Recognize that complex reactions may have fractional orders or orders that don't directly reflect stoichiometry.
-
Use Kinetic Data Effectively: Analyze kinetic data carefully to identify rate-determining steps and interpret reaction mechanisms.
Final Conclusion: Wrapping Up with Lasting Insights
Understanding reaction order and molecularity is fundamental to mastering chemical kinetics. These concepts, though distinct, are interconnected and essential for comprehending reaction rates, designing efficient processes, and developing new materials and technologies. By combining experimental data with theoretical understanding, a deeper comprehension of reaction mechanisms and their implications can be achieved. The ability to accurately predict and control reaction rates is vital across various scientific disciplines and industrial applications.
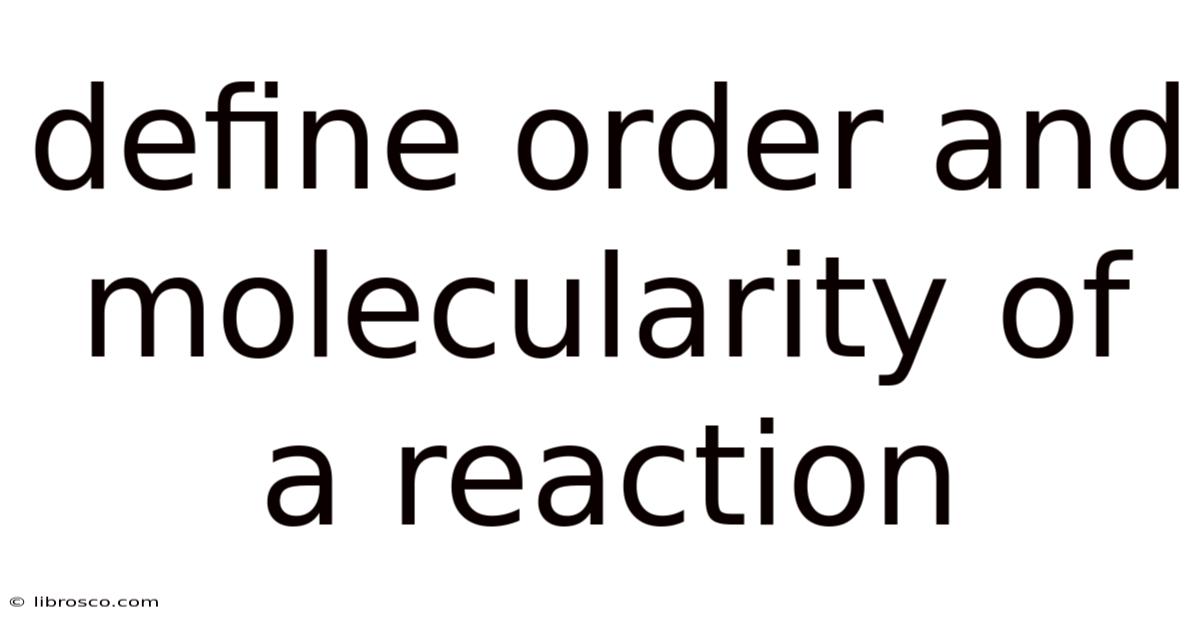
Thank you for visiting our website wich cover about Define Order And Molecularity Of A Reaction. We hope the information provided has been useful to you. Feel free to contact us if you have any questions or need further assistance. See you next time and dont miss to bookmark.
Also read the following articles
Article Title | Date |
---|---|
Bootstrapping Self Funding | Apr 21, 2025 |
Mosaic Theory Meaning | Apr 21, 2025 |
Define Order In Biology | Apr 21, 2025 |
What Is Income Tax Accounting | Apr 21, 2025 |
What Is Deferred Tax Accounting | Apr 21, 2025 |